Researchers have made a groundbreaking discovery by demonstrating that the complex behavior of chaotic quantum systems can be described using the principles of fluctuating hydrodynamics, a theory typically associated with classical systems. This finding could pave the way for a better understanding of the intricate dynamics of quantum many-body systems.
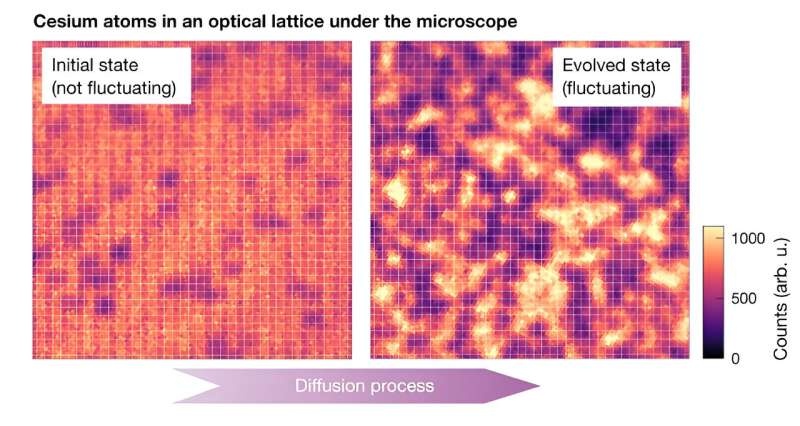
Crossing the Quantum-Classical Divide
Because the constituent particles of quantum systems can exhibit profoundly weird, counterintuitive phenomena like entanglement, they behave in ways that are profoundly different from ordinary matter at human scales.
Simulating the behavior of these complex quantum systems has been, by tradition, an insurmountable challenge because of the vast number of particles and their interconnectivity that must be modeled.
In this context the study faces a challenge in quantum mechanics but still researchers have managed to circumvent it by using hydrodynamics (an approach usually suitable for classical systems), called fluctuating hydrodynamics (FHD).
The main finding is that a state of local thermal equilibrium among its particles can emerge in all these chaotic quantum systems because the particles interact in a specific systemic way. This property effectively turns the system into a continuous density field following simple differential equations that researchers can use to describe large parts of its macroscopic behaviour, instead of needing to keep track of each singe particle movement.
High Resolution Quantum Gas Microscopy Reveals Link Between 1D and 2D Physics in the Quasi-2D Regime of an Optical Lattice
The researchers turned to the quantum gas microscope — a highly tunable experimental setup that enables probing of chaotic quantum systems.
With this, they could trap ultracold cesium atoms in an optical lattice, eventually forming a quantum many-body system where the atoms could interact.
The researchers take high-resolution snapshots of the system, and follow how fluctuations evolve over time as the atoms move around and interact, settling to a state where temperatures become equal for all particles.
By comparing the growth rate of these fluctuations with predictions of FHD theory, the team could confirm that the macroscopic description from chaotic quantum systems is indeed captured by this theoretical framework and even measure directly for the first time a crucial constant in such dynamics, named diffusion constant.
Conclusion
The results obtained by this research team are groundbreaking and have important repercussions in the study of chaotic quantum systems. In doing so, the researchers have shown that fluctuating hydrodynamics — a venerable classical theory of physics (a branch of physics which does not admit to a quantum description) — can be applied to this more exotic and strange system, thereby providing new tools for analyzing these systems in their complex nonequilibrium behavior.
Besides bridging the gap between quantum and classical physics, this discovery offers a new way of studying chaotic quantum systems—from ultracold atomic gases to exotic materials. With further research into the limitations and applications of this method, we will continue to uncover the intrinsic nature quantum many-body dynamics.