Explore the groundbreaking research on how heat behaves in tightly confined spaces, and its implications for a wide range of applications, from energy efficiency to biomedical devices.
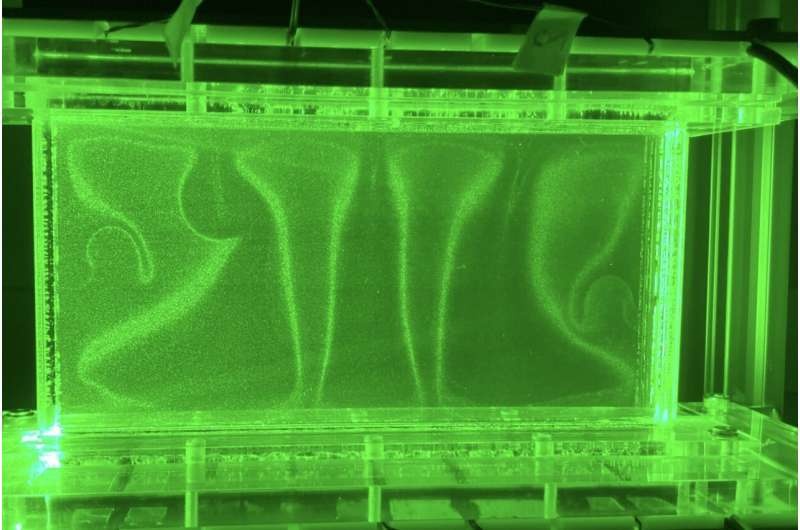
The counterintuitive truth about air fryers
Air fryers have been making waves in culinary circles, touting a healthier way than the deep fats of yore for frying delicious treats. However, little did we know that the underlying technology of these current meal contraptions is using a heating mechanism simple yet found in nature?
Air fryers work, using the process of convection heat — and we use that term like Hugo Ulloa, a fluid dynamics scientist from the University of Pennsylvania who confirmed the science behind one particular air fryer design or another. For instance, this is the reason that water being heated from below in a pot will cause movement in all of the water, since the less dense warmer bottom layer pushes upwards.
Convection is indeed a familiar process within the relatively wide-open realms of our atmosphere and oceans, but it has been left largely to mystery how heat moves in super-confined spaces like the small chambers inside air fryers, Ulloa and his colleagues find. The results of their groundbreaking research, published in Proceedings of the National Academy of Sciences this week, illuminate how heat and fluids operate in these extremely confined settings.
Revealed the Secret of Confined Convection
To study convection at these smaller scales, Ulloa and his team — which also includes postdoctoral researcher Daisuke Noto — imagined a set of experiments that could be done on a Hele-Shaw cell. Such a device is based on two parallel plates whose lower plate heats the inner fluid from below and the upper cools it down; these two plates are separated by a small vertical gap for convection.
Through control of the gap size and temperature gradient, the researchers could then observe how heat and fluid flow depend on the dimensionality of confinement. And what they found was incredibly interesting.
As the system approached compression, thermal plumes began to form — small mushroom-shaped forms that separate from the base and are a key part of convection. Depending on their size relative to the gap, they may either grow freely in three dimensions or be restricted to a two-dimensional flow.
According to Ulloa, this changing between three-dimensional and two-dimensional flow is major in the difference of heat transfer. Hence if the gap was much larger than the natural width of plumes, each individual plume would freely grow disjointed in 3D to give rise to high but poorly efficient heat transfer. Yet when the gap was narrowed, these thermal plumes were squished and became two-dimensional flows—if you will—with more of the energy being used to heat transfer effectively.
Conclusion
The pioneering research conducted by Ulloa, Noto and the members of their lab has led to a new insight into heat dissipating behaviour in finite volumes with wide-ranging consequences. Insights derived from the new research may lead to a host of advancements in environmental and engineering applications — ranging from more efficient harvesting of geothermal energy, to enhanced cooling systems for high-performance computers. With the researchers diving deeper into how substances mix in these tight quarters, who know what other interesting movements we might discover that could revolutionize our understanding of both heat transfer and fluid dynamics.