A new study uncovers the intricate mechanisms behind the dynamic slowdown of water at low temperatures, shedding light on the glass transition in liquids. Explore the role of dynamic disorder and the complex interplay of molecular motions that govern this intriguing phenomenon.
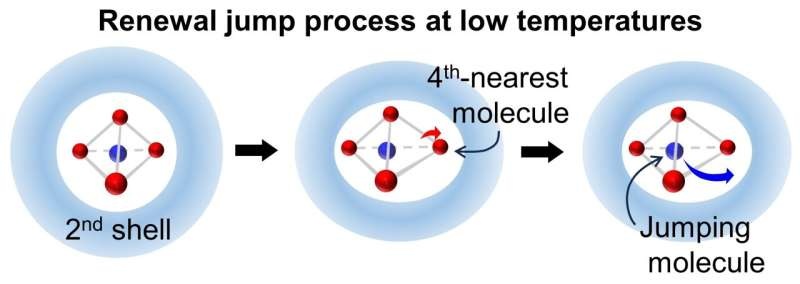
Cracking the problem of supercooled water
Water is an amazing material, and its characteristics have fascinated scientists for over a millennium. A particularly fascinating idea is the supercooling process, whereby water remains in liquid form despite being cooled below its normal freezing point to not freeze.
Now a new study by an analyst at the Institute for Molecular Science has sought to reveal the hidden dynamics that intertwine to produce this curious response. Leveraging molecular dynamics simulations, the study investigates the microscopic origin of the substantial slowdown in dynamics upon cooling supercooled water.
The headline result is that the slowdown appears due to dynamic disorder. Dynamic disorder, i.e. the competition among slow variables such as fourth-nearest oxygen atom displacement and jump motions of water molecules; As the temperature gets lower, these competing factors get more complicated causing the water molecules to be stuck in frozen extended, stable low-density regions.
Molecular Movements: The Complexity of the Choreography
The results of the study shed much-needed light on the complex choreography of molecular motions in supercooled water. At lower temperatures, molecular motion slows and becomes sporadic as the water molecules are confined inside these large, low-energy domains.
The water molecules become increasingly cooperative, and this new change in molecular behavior comes from that. As the temperature drops, the molecule-molecule interactions become more correlated, and with that jump, dynamics become more complex and dimensional.
The fourth-nearest oxygen atom displacement, a slow variable that competes with the jump motions of the water molecules, is referred to by the researchers as a collective coordinate. Essentially, this is the general displacement beyond the first hydration shell and it has a significant effect on the global dynamics of our system.
This insight into the microscopic gears is a crucial part of solving the mystery behind how glass transition takes place in liquids. The tools and findings developed in this work can be used for many materials, giving important information on the role of slow dynamics in generating kinetically trapped glassy states.
Conclusion
By investigating the behavior of supercooled water at individual atomic lengths and time scales, this study also reveals an unprecedented role of two distinct kinds of dynamic disorder in the sluggish molecular motions governing super dynamicity. Through their observation of this complicated interplay between slow variables and jump motions the researchers have set the stage for a new level of description around glass transition phenomena in liquids.
The applicability of the methods and insights from this work lies in a wide array of materials and physically relevant systems well beyond just water. By mechanically forcing them beyond their breaking points, the study opens the door to new research into the intricate workings of a variety of other complex fluids proteins and also their applications in transition glass processes.