Researchers at Université Grenoble Alpes have made a significant breakthrough in the development of more stable superconducting qubits, the building blocks of quantum computing systems. Their study, published in Physical Review Letters, demonstrates the direct measurement of a subtle current phase relation, known as the sin(2φ) current phase relation, in a graphene-based superconducting quantum interference device. This discovery paves the way for the creation of quantum bits that are less prone to decoherence, a major challenge in the field of quantum computing.
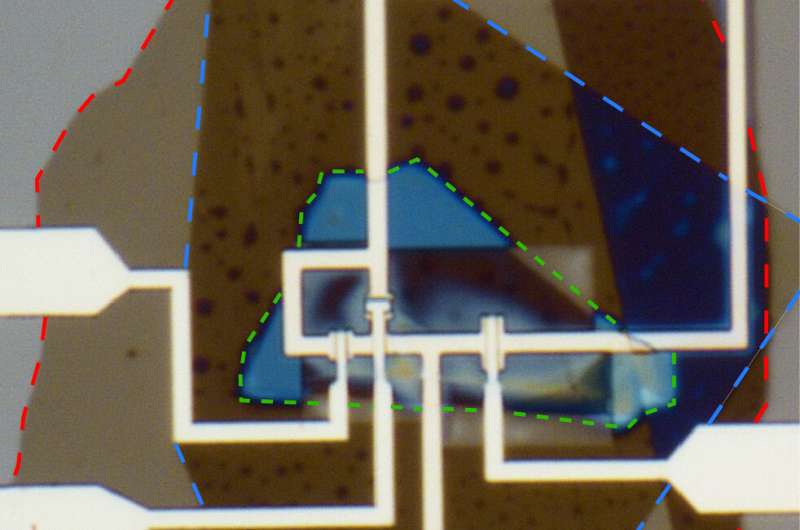
The Sin(2φ) Phenomenon Revealed
In recent years, quantum physicists and engineers developed an advanced system in the field of quantum computing. Nonetheless, achieving a quantum advantage relative to classical computation as well as confirming reliable operation conditions require devices with new building blocks and understanding the underlying physics.
The current phase relation in a graphene-based superconducting quantum interference device (SQUID) was directly measured by a team comprising researchers at Université Grenoble Alpes and senior author Julien Renard. The measurement is significant as it can be used to design superconducting quantum circuits with customized properties, which could yield more consistent and coherent superconducting qubits.
The Groundbreaking Experiment
In the team’s experiment, for example, they observe voltages from their graphene-based SQUID as a function of externally manipulable parameters, such as an external magnetic field. This approach was used to both control and probe the instantaneous phase relationship of the Josephson junctions in their device, which represents a significant advance over previous experimental studies on this system.
Renard: A superconducting interference device allows to vary the phase by means of the magnetic field. In turn, The measured signals enable to reconstruct current for different impedance aspect ratios. This is how we would be measuring the phase relationship of the device right now. The results of the experiment demonstrate that their device acts as a sin(2φ) element, which means that its current-voltage characteristics exhibit a pattern described by sin(2φ) rather than the typical sin(φ) behavior observed in “conventional” Josephson junctions.
Bridging the gap to better qubits
The experimental methods used by the team, including the unique phase relationship it discovered in its device, could soon find use in improving quantum computing technologies. The researchers hope to use the sin(2φ)-current phase relation to create new kinds of more robust quantum bits for use in ways that impede decoherence, a huge problem in quantum computing.
Renard added, “We demonstrated that by assembling two graphene Josephson junctions into a superconducting quantum interference device we can control the interference effects between Cooper pairs with a magnetic field in order to obtained a sin(2φ) current phase relation. This kind of graphene superconducting quantum interference device might serve as the key element in the creation of a new generation of topologically protected qubit. So, we will strive to find the right circuit geometry for that quantum bit now”