Scientists have shown that tweaking the momentum of light can make silicon a better semiconductor. Future applications of this finding are going to transform solar energy conversion and optoelectronics.
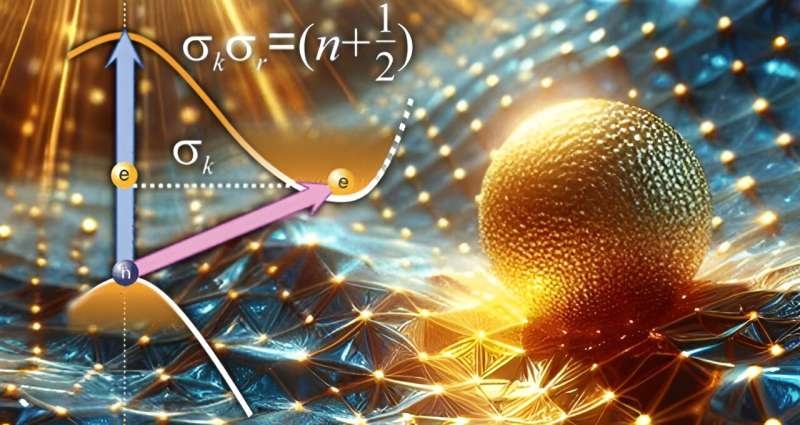
Resetting the Text-Book of Light-Matter Interactions
For many years, light-matter interactions were understood to be properties of material itself — meaning that the optical and electronic property of a natural material is well established. Yet, further work at UC Irvine that turns this idea on its head.
By carefully choosing the momentum of incoming photons, the researchers were able to show that light could adopt an entirely different personality when interacting with matter. This phenomenon of photon generation is a direct manifestation of the Heisenberg Uncertainty Principle; i.e., light confined in nanoscale dimensions gains momentum in excess to that corresponding with free-space photons by over three orders of magnitude.
Because of this huge momentum kick from the photons, electrons can do previously disallowed diagonal transitions. In other words, these momentum-enhanced photons make it possible to “tilt the textbook,” said the researchers, as this allows for changing of both energy and momentum states of electrons. By enabling the tunable absorption and light emission characteristics of matter, this means that an entirely new realm of opportunities has opened up.
Conversion of Silicon to Direct Bandgap seminconductor
Among the examples in the researchers’ findings is pure silicon becoming a direct bandgap material from being an indirect one, and silicon is ubiquitous and important semiconductor. A team of researchers has succeeded in solving a basic problem unsolved to date with silicon, the second most abundant element in Earth’s crust and the backbone of modern electronics: its poor ability to absorb light.
With the indirect bandgap of traditional silicon, it requires phonons (lattice vibration) to be present to facilitate electronic transitions. Silicon is hardly optically active because the photon, phonon, and electron does not happen to collide at the same space time. For example, silicon-based devices (including solar panels) rely on bulky layers of the material to absorb the maximum amount of sunlight.
The researchers introduce a completely novel route to achieve diagonal transitions by coupling it with particles of momentum-enhanced photons, which effectively changes the band character of silicon from indirect to direct without physically modifying the material in question. When silicon undergoes this dramatic metamorphosis, its light absorption capability is boosted by several orders of magnitude. The advance could lead to significantly thinner silicon wafers and solar cells capable of serving the same purpose as conventional, bulky silicon technologies at a small fraction of the cost.
Conclusion
UC Irvine’s pioneering work shows how the properties of light can be combined with those of an object in such a way as to realize the promise of an existing material. Researchers have broken new ground in the development of a type of transistor — technologically crucial for energy conversion — that is fast and efficient enough to be potentially used in a newly emerging class of electronic devices. Scientists are exploring these devices even for “neuromorphic” applications, which seek to mimic the connections between neurons in the human brain. This unsurpassed discovery has the potential to revolutionise solar cells and create a significant impact on the cost and efficiency of these devices, as well as a host of other novel applications. This is a potential game-changer in the field of silicon technology, at a time where an exponential growth in transitioning to renewable energy sources is required globally to alleviate the rapidly worsening impacts of climate change.