Researchers have made a groundbreaking discovery about the molecular mechanisms that determine the spiral shape of certain bacteria. This finding could lead to new ways to influence bacterial cell structure and potentially combat pathogens. The study, published in Nature Communications, focuses on the photosynthetic bacterium Rhodospirillum rubrum and its unique spiral shape, which is crucial for its environmental adaptability and biotechnological potential.
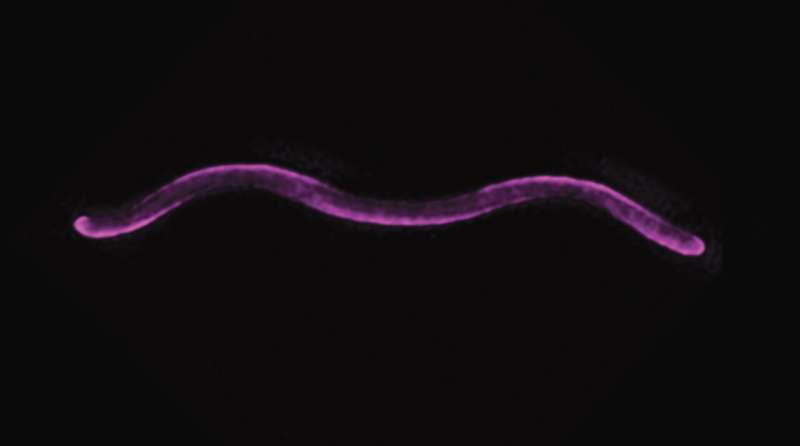
Unraveling the Molecular Secrets of Bacterial Shape
Bacteria show an unbelievable repertoire of shapes, from the classic rod-shaped Escherichia coli to a wide range of curved and spiral-shaped forms such as Vibrio cholerae or Helicobacter pylori. Scientists have been puzzled about how such cell shapes arise, because they are so important for the bacteria to stick, swim and even harbour infection.
An international team of researchers led by Martin Thanbichler, Professor at the University of Marburg in Germany has now elucidated how the helical shape of the photosynthetic bacterium Rhodospirillum rubrum is generated. This species is a food for wildlife and it has good biotechnological capacities: this species can use carbon monoxide, produce nitrogen by fixation and they also synthetize hydrogen (which could be obtained from human waste), building blocks to obtain bioplastics.
Harnessing Bacterial Shape for Environmental and Biotechnological Applications
When the researchers studied porins, for example – channel proteins long believed to exist solely on the outer membrane of bacteria where they form clustered openings through which nutrients pass from near a cell’s surface to nearer its center– they found them in an orderly helical array, running straight up and down along the outside curve of Rhodospirillum. This pore structure, which is integrated into the cell wall by a close association with a second protein, PapS.
Lastly, the cells became completely straight when we prevented PapS from binding to the porins suggesting that its presence is necessary for formation of the spiral shape. Martin Thanbichler: “The porins appear to have been reprogrammed at the molecular level for an additional function that is orthogonal to their role in nutrient turnover.” PapS and the other protein stop a molecular machine — “a nano-wheel” — from moving, legacy of a circle line on the cell wall. Which introduces new material to the existing wall of a cell and promotes cell elongation.
Uncovering the Role of Porins and Lipoproteins in Bacterial Curvature
In rod-shaped bacteria like E. coli, this elongation machinery is evenly distributed over all areas of the cell, resulting in a straight shape. In contrast, the elongation machinery appears to be partially immobilized at the outer curve in RSP-Fab complexes with the helical Porin-PapS structure that creates a molecular cage in Rhodospirillum rubrum. This creates a local increase in cell elongation at the Porin-PapS structure, which then causes the cell body to bend into spiral.
“R. rubrum now provides us with a way of changing the cell shape,” says study first author Sebastian Pöhl. It will allow us to investigate under which circumstances the helical cell shape is beneficial for bacteria in their natural environment. This could lead to valuable information on how changes in cell shape contribute to the colonization of ecological niches, the establishment of symbiotic relationships with plants, or a disease. These findings are likely relevant to all relatives of Rhodospirillum that have this curvature, and it will be interesting to determine if this mechanism is used among other more anatomically asymmetrical (i.e., crescent-shaped) bacterial groups as well.